Modelling pneumatic hopper discharge of fine bulk solids with the EDEM-AcuSolve coupling
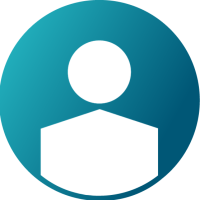

Pneumatic conveying is commonly employed for the transportation of bulk solids in industrial manufacturing processes due to its safety, versatility and ease of automation. However, challenges such as plug flow, agglomeration and blockages are present in pneumatic conveying systems and lead to a lower degree of control, higher maintenance requirements and reduced product quality. These problems are particularly common for fine bulk solids and can sometimes be traced to hopper feeders of the pneumatic line where arching and ratholing result in intermittent discharge and pressure surges [1].
Developing an understanding of the complex interaction between operational parameters and bulk solid properties that gives rise to these phenomena can result in improved process operation. However, relying on physical experiments alone for this purpose may be time consuming and expensive making numerical modelling a useful component of the study that can reduce costs and provide additional insight.
Numerical modelling and analysis of multi-phase systems such as a pneumatic conveying line can be carried out using a two-way coupled CFD-DEM modelling approach, whereby the bulk solid flow is resolved in Altair EDEM and the air flow is resolved in Altair AcuSolve. For further details on setting-up a coupled EDEM-AcuSolve simulation please review our E-learning and tutorials.
The modelling of a pneumatic hopper feeder system was carried out in collaboration with Novo Nordisk, with the aim of relating the system performance to the pressure drop and the powder flowability. The system consists of an axisymmetric hopper and a horizontal pipe with an air valve downstream of the hopper as shown in Figure 1.
Figure 1 A two-waycoupled CFD-DEM model of a pneumatic hopper feeder system
The bulk solid is modelled in EDEM via a meso-scopic approach, in which numerical particles of an intermediate scale between the physical particle scale and the scale of the system of interest are used to achieve practical computational times. The effect of particle shape is accounted for using the multi-sphere approach and the Edinburgh Elastic-Plastic-Adhesive contact model is used to model the visco-elastic-plastic-cohesive behaviour of the powder [2]. The bulk solid model is automatically selected from the EDEM Powders Database using direct shear test measurements [3] for Micro-Crystalline Cellulose (MCC) as search criteria. The particle to hopper wall interaction parameters are calibrated against wall yield locus measurements of MCC sliding against stainless steel.
The Euler-Lagrange methodology is used for the fluid and solid phase coupling in which the fluid domain is discretized using an unstructured tetrahedral mesh in AcuSolve. An unresolved CFD-DEM bi-directional coupling approach is used for considering the effect of the motion of the particles on the fluid flow. In this approach, the volume averaged Navier-Stokes equation is used for the fluid phase through the local averaging of fluid-particle interaction force and the solid phase volume fraction. The Gidaspow correlation is used for calculating the drag force on the particles.
The predictive accuracy of the numerical model is validated against experimental measurements of the mass flow rate of micro-crystalline cellulose in the pneumatic hopper system.
Validation cases with a fully closed and fully opened air valve are considered in order to cover the pressure drop extremes in the system. For the closed valve case, the air opening is blocked completely and the pressure at the outlet is varied while in the open valve case, a constant air flow rate, obtained through experimental measurements, is applied at the air valve inlet. A comparison of the simulation and experimental results is shown in Table 1 and the computed air velocity and particle volume fraction of a closed valve and open valve case are shown in Figure 2 and 3 respectively. A good quantitative agreement is observed between the predicted and measured mass flow rates for both cases, while the corresponding simulated bulk solids flow patterns qualitatively agree with experimental observations. Taken together these observations are indicative of the validity of the modelling methodology.
Valve configuration | Pressure drop (kPa) | Transferred mass (in 3 sec) | |
Experiment (kg) | AS-EDEM (kg) | ||
Closed | 20 | 2.126 | 2.538 |
Closed | 22 | 3.303 | 2.727 |
Closed | 23 | 3.088 | 2.645 |
Open | 2 | 0.466 | 0.375 |
Table 1 Comparison of the transferred powder mass between the simulation and experiment
Figure 2 Velocity magnitude and solid volume fraction contour plot for closed valve case at 23kPa pressure drop
Figure 3 Velocity magnitude and solid volume fraction contour plot for open valve case at 2kPa pressure drop
The numerical model of the closed valve case is used to perform a parametric analysis with the aim of relating the applied pressure drop and bulk solids flowability to process performance.
To this end, the discharge of bulk solids of two flowability extremes is simulated at a wide range of pressure drops. The predicted evolution of the mass flow rate of the low cohesion material at varying pressure drops is shown in Figure 4 and the effect of cohesion on the discharge at a fixed pressure drop is shown in Figure 5
Figure 4 Predicted mass flow rate at varying pressure drops for a material of low cohesion
Figure 5 Predicted mass flow rate at 20kPa pressure drop for materials of two cohesion extremes.
The results reveal a transitional flow behaviour characterised by a shift from a continuous flow to the intermittent arching behaviour shown in Figure 6. This is as a result of a complex interaction between outlet pressure and powder flowability, whereby the propensity for arching increases with the pressure drop due to an increase in the consolidating stresses in the powder bed and a corresponding increase in bulk material strength in the region of the arch.
The intermittent arching results in a non-linear relationship between the mass flow rate and pressure drop with the mass flow rate increasing sub-linearly with pressure drop.
The arch stability increases with bulk cohesion, resulting in a highly discontinuous flow behaviour for cohesive materials even when discharging under low pressure drops.
A significant localised increase in the consolidating stresses in the region of the outlet can be observed downstream of an arch collapse as shown in Figure 6 and this may result in unwanted agglomeration and material build-up. This is consistent with experimental observations of material build up in the region of the pipe elbow.
Figure 6 Intermittent arching behaviour during discharge of a low flowability bulk solid.
The results suggest that simply increasing the pressure drop may not be a remedy for poor flow in a pneumatic hopper feeder system and the relationship between the pressure drop, consolidating stress and powder flowability needs to be carefully considered.
References
[1] Konrad, K. (1986). Dense-phase pneumatic conveying: A review. Powder Technology, 49(1), 1–35. https://doi.org/10.1016/0032-5910(86)85001-X
[2] Thakur, S. C., Morrissey, J. P., Sun, J., Chen, J. F., & Ooi, J. Y. (2014). Micromechanical analysis of cohesive granular materials using the discrete element method with an adhesive elasto-plastic contact model. Granular Matter, 16(3), 383–400. https://doi.org/10.1007/s10035-014-0506-4
[3] ASTM Standard. (2008). Standard shear test method for bulk solids using the Schulze ring shear tester. ASTM International, Www. Astm. Org, i, 1–27. https://doi.org/10.1520/D6773-16.2