Extend Your Range with Digital Twins for Thermal Battery Management
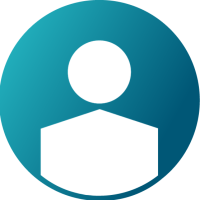

Laptops, smartphones, electric vehicles, power tools, or your toothbrush: mobile applications like this need stored mobile energy to fulfill their tasks. For larger systems, like battery electric vehicles, the mobile energy requirements grow profoundly, and energy storage capacity plays the starring role in broad acceptance of e-mobility. But what is so special about batteries in mobile applications and what makes a good battery design? In many electric vehicles, the battery is the most valuable component, which means battery development and operational performance are essential to get right. For this article, I’d like to talk about a critical area of concern for e-mobility developers – battery internal temperature, which heavily impacts battery and vehicle efficiency. You might ask, “Isn’t a battery pack just a bunch of battery cells?” Let’s look at some of the details and find out.
Battery Challenges for E-Mobility
Delivering compact and lightweight designs are well-known goals in vehicle development. Size and vehicle weight need to be constrained if the target is a streamlined, dynamic, and long-lasting driving experience. The highly dynamic operating conditions of an electric drive also need to be considered in relation to the battery system’s space and weight limits so that drivers can know what range to expect under different types of driving conditions. Along with space and weight boundaries, cooling is another aspect to consider, especially since, compared to electric machines or electronics, the battery’s long- and short-term performance as well as the vehicle’s safety are much more dependent on the cell temperatures.
Moreover, heat generation and its effects on the battery are complex and require expertise from different physical domains such as electrical, structural, thermal, fluids, and controls. This leaves us with several levers to pull and much more potential for increasing efficiency if we can take all these domains into account at the higher system level.
From Cell to System
A single battery cell’s capability to conduct electric current and store electric energy is mainly defined by the microstructures of the anode and cathode materials as well as the cell’s size. However, the inner structure of the cell also defines how the generated heat is propagated to the outer layers and the cell’s housing. Knowing about the heat propagation from inside to outside of the single cell, we can design module configurations and cooling concepts efficiently. Heat propagation inside the battery module and the pack is mainly between the cells and possibly includes the damping materials via conduction, advection, convection, and radiation. The connecting busbars also heat up because they conduct electric current. To solve this, optimization via simulation can help determine the optimal temperature distribution and reduce material costs.
We draw vast information about cells, sub-modules, modules, and complete battery packs by leveraging virtual prototypes with different fidelity levels. Combining electro-thermal losses, including their specific localization in the pack, and the heterogenous material mix of the pack makes this a true multiphysics evaluation that can provide critical insights for informing design decisions.
Avoiding Battery Damage
Uncontrolled heat generation in a single cell can be triggered by a crash, extensive external heat, or overcharging. These external influences will at some point lead to short circuits inside the cell structure, instigating the cell to heat up. The extensive heat of one cell can then potentially propagate through the battery pack and heat up other cells, a scenario called “thermal runaway,” which often leads to harmful consequences including chemical fires.
Such runaway scenarios can be avoided with detailed insights into the battery structure by leveraging virtual prototypes. Using virtual sensors from models can help predict cell temperatures and optimize the cooling system and strategy to increase battery safety. When performing structural analysis in crash situations, it is essential to homogenize models from high detail and a high number of finite elements to lower-element numbers so that the simulations run in an acceptable time.
Digital Twin Technology for Batteries
Why do we need Battery Digital Twins? Knowing more about temperature distribution and how temperature is going to change in specific scenarios or drive cycles is crucial for spending the stored battery energy efficiently and avoiding damage. Leveraging that knowledge in the battery models can provide virtual sensor data not only as input to the battery design but also to the battery management system (BMS) while the battery is in operation.
Despite the challenges of linking the different domain sub-models correctly, we can compare live sensor data from batteries with simulated data – in real-time. This process involves shifting from a high-fidelity, computationally expensive, model to an efficient model, preferably for real-time controller applications, that runs in milliseconds. Is that even possible? Reducing details of simulation models is possible, the secret is knowing how to reduce while preserving the important information and retrieving accurate results while increasing simulation speed. To achieve this, we use an Altair-developed, reduced-order modeling approach leveraging machine learning. Combining these workflows with data analysis delivers a very helpful Battery Digital Twin for mastering the challenges of thermal management.
Happening Now
- Learn more about Altair's digital twin applications in our upcoming webinars.
- Reserve your spot at ATCx AI for Engineers 2024 and join us for the presentation: The State of Generative AI in the Enterprise: Now Decides Next with Stavros Stefanis, Principal Partner, Deloitte Consulting, and Jeff Glueck, Senior Vice President, Strategic Relationships, Altair.
The Future
Altair provides the most comprehensive digital twin solutions, comprising best-in-class technologies that can be seamlessly integrated into established workflows. Beyond delivering software, Altair has domain experts at your service to help you achieve your goals more efficiently!