DEM Analysis of Lithium Ion Battery Electrode Structures Under Different Calendering Conditions
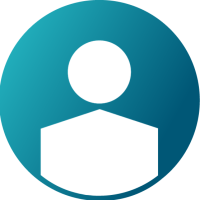
This work was produced by Dr Ruihuan Ge, Postdoctoral Research Associate at The University of Sheffield, as part of the NEXTRODE project funded by the FARADAY Institution.
Lithium-ion batteries are widely used as energy storage devices. However, the relationships between manufacturing parameters, electrode microstructure, and subsequent battery performance are not well understood. The electrodes contain many different materials, spanning different phases, including active material (AM) particles, carbon binder domain (CBD), and the pore space in-between. This is where our NEXTRODE project (run at the University of Sheffield and funded by the Faraday Institution [1]), comes into play as it sheds light into the next generation lithium-ion battery electrode manufacturing and microstructure design.
Let us imagine two very large rollers (usually 50-200mm radius) that compact a very thin electrode (usually 50-200μm thickness). This is an essential step in manufacturing lithium-ion battery electrodes, which is called CALENDERING! During calendering, the electrodes are compressed to tailor the electrode porosity and improve the battery performance. During this process complex physical property changes occur that we don’t yet understand; hence numerical modelling of the calendering process is gaining much attention. However, the high-fidelity simulation of battery microstructure evolution during calendering has been demonstrated to be an important but still challenging task.
In our recently published work [2], Discrete Element Method (DEM) with a bonded particle model was used to investigate the evolution of electrode microstructure under varying calendering conditions. For the first time, using accurately defined structural properties, DEM simulations of electrode calendering are validated using X-ray tomographic data. As shown in Figure 1, a subdomain of NMC 333 electrode structure was chosen from the database [3] and imported into DEM simulations. Inter-particle bonds were generated between AM particles to describe the mechanical response of the CBD phase within electrode structures (Figure 1 c).
Figure 1 Electrode structure generation used in DEM.
Electrode structural properties including porosity distribution, specific surface area and tortuosity factors were studied and compared with corresponding tomography scans. The porosity changes in DEM simulations were found to be in good agreement with the experimental data from tomography scans at respective stress levels (Figure 2 a). Calendering can affect the particle contact distributions and stress distributions within an electrode. As shown in Figure 2 (b), contact force chains are formed and more homogeneous inter-particle contacts can be generated with increased calendering levels. The transport properties are further evaluated and shown in Figure 2 (c). Tortuosity is an important parameter as it links the morphology of the pore space of a lithium-ion battery to the effective transportation of the ions. In other words, tortuosity regulates the charging of the battery. For evaluating the tortuosity factor, the pendular ring-shaped CBD phase was generated. For the first time, the results show the tortuosity of a calendered electrode can be predicted by combining DEM simulation and a binder phase generation algorithm (Figure 2 c).
Figure 2 Mechanical and transport property analysis by DEM.
Our research work demonstrates, for the first time, the feasibility of combining X-ray tomography and DEM to analyse electrode structure evolution during calendering. This offers the opportunity to optimise electrode structures with desired mechanical and transport properties in the future. By using Altair EDEM, our research group is demystifying the complex physical properties of battery electrodes during manufacturing, e.g., elastic-plastic mechanical properties, and non-spherical shape effect.
This newly published paper is accessible to all and can be downloaded through the following link: https://www.sciencedirect.com/science/article/pii/S0032591022002601.
References
[1] https://nextrode.web.ox.ac.uk/home.
[2] Ge, R., Cumming, D.J. and Smith, R.M., 2022. Discrete element method (DEM) analysis of lithium ion battery electrode structures from X-ray tomography-the effect of calendering conditions. Powder Technology, 403, p.117366.
[3] Ebner, M., Geldmacher, F., Marone, F., Stampanoni, M. and Wood, V., 2013. X‐ray tomography of porous, transition metal oxide based lithium ion battery electrodes. Advanced Energy Materials, 3(7), pp.845-850.