Real-Life Applications of Drivetrain CFD
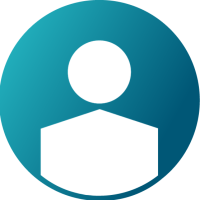
Finite Volume (FV) Computational Fluid Dynamics (CFD) have been around for long enough that we probably do not have the need to go through its entire history, nor really explain how it revolutionized engineering in every single industry branch out there. From aerospace, automotive and marine industries to food processing, electronics and medical branches – FV CFD got almost everywhere. Almost.
After close to 40 years of industrial growth and established market domination, it became clear that solving certain types of CFD problems cannot be solved easily just by throwing more cores at the problem. I have once heard from a head of research of a major company saying, “We have tried doing this problem with 15,000 CPU cores and it still took a week. We need a change of paradigm.” Not everyone has a super-computer at hand.
One subset of CFD problems which all Eulerian CFD methods (finite volume, finite element) have trouble with can be considered a niche but permeates many of the mainstream industries – it is drivetrain oil and thermal management. Present in all vehicles be it land, sea or air, with ever increasing demand for efficiency, weight reduction and with increasing power density – oil supply and its cooling role in drivetrain are becoming ever more important.
Classic Eulerian methods are ill-equipped to handle these cases efficiently. High-resolution mesh requirements to reduce interface diffusion, mass conservation trouble, cumbersome pre-processing in combination with various “band-aid” solutions (e.g. overset mesh) and resulting high computational cost of such potential simulations is not something that fits into a modern, high-paced design process. To be clear, no one is doubting the ultimate fidelity of the Eulerian CFD methods, but in the case of drivetrain oiling FV methods clearly face a number of challenges that cannot be solved with any of the “off-the-shelf” tricks that Eulerian CFD has to offer.
In the opposite corner, there are the rising stars of the CFD world – the Lagrangian CFD methods, led by the Smoothed Particle Hydrodynamics (SPH) method. In the broad spectrum of Altair’s products, the main SPH representative is Altair nanoFluidX. With perfect mass conservation and convection, razor sharp fluid interfaces, highly parallelized, powered by Graphical Processing Units (GPU’s), and with vastly easier pre-processing – nanoFluidX’s SPH method appears like a tailored solution for drivetrain oiling.
No, SPH does not have all the bells and whistles of a FV CFD method, but for this use case – it does not need them. What it does have is a clear performance edge and greatly simplified workflows to fit into a modern engineering design process and provide critical feedback to its users. The reduction in Turn-Around Time (TAT) is an order of magnitude when compared to anything Finite Volume.
I want to pause and dwell a bit here with the previous thought. This is the wrong place to spark a discussion on peak accuracy abilities of FV and SPH methods. I have seen both methods do amazing things hitting similar levels of fidelity when pushed to the brim of method’s abilities. This is not about that. Real-life drivetrain CFD requires sufficient levels of accuracy, but just as importantly, it requires TAT (from raw CAD to results) of a few days, and ideally of a few hours. FV cannot deliver this in a realistic environment. SPH and thus Altair nanoFluidX can.
In engineering, and probably more generally – life, the golden rule of “20% of effort, 80% of the results” goes a long way. Drivetrain oiling using SPH method is more like 10-90. It takes a fraction of the time and effort (of the FV approach) to give sufficiently good, and often excellent, results. This is what real-life drivetrain CFD is all about.
Digging deeper into the technical aspects – there is a lingering question of single vs. multiphase simulations. It is often the case that the air volume inside the drivetrain is significantly larger than the oil volume. This is to say that if you neglect the air in the simulation, the computational cost will go significantly down. To add to that, when running in single phase, several numerical parameters work in your favor and that only makes things faster.
While there are certainly conditions and operating points where single phase simulation can give satisfactory results (e.g. low RPM operating points), it is ultimately the engineering judgement that has to make the call what is acceptable.
In our experience, if your gears are hitting 10 to 15 m/s of tangential velocity – windage effects already start to play a role. At 20 or 25 m/s of tangential velocity, windage is already strong and it is very questionable if it should be ignored.
So, is there a universal recipe to how to approach this single vs. multiphase conundrum?
If we observe a design process of a subsystem (e.g. an electric vehicle gearbox or an e-motor), we can try to isolate three stages – early design, where we have a vague idea of the design and we would like to experiment with a large number of variations to quickly eliminate the worst ones. This is where we can use single phase simulations. Each simulation would take a few hours even on modest hardware resources.
The middle stage of the design, where we have down-selected 2 or 3 designs. This is where multiphase simulations can be introduced to increase the overall fidelity of the comparison, although it may not be necessary to run the complete matrix of operating points to discern among the designs.
Finally, the late design stage, where we have selected the best design and we are now proceeding to understand its intricacies. We do so by adjusting the numerical parameters for higher fidelity and covering more operating points under various conditions.
As we go through the process from early to late design stages, we increase the cumulative number of simulations and gain better understanding of the oil behavior, therefore decreasing the risk that the design would fail on the actual prototype test stand or out in the market (See Figure 1 below).
Figure 1: Design cycle of a drivetrain component. It starts with single phase, low-fidelity simulations and we progressively increase the fidelity as we narrow down the details of our design.
The goal is not to replace the experiments completely – the goal is to make only one experiment and be confident that the chosen prototype will perform as intended. This confidence comes from tools like the Altair nanoFluidX performing CFD simulations throughout the design process.
It is therefore important to note that it is not enough to just have the right tool – it is equally important to know how to use that tool from a methodological point of view. It is not just about running a sweep of simulations – it is important to understand the numerical and physical nuances and define ahead of time what are we expecting to see from the simulation. Using engineering intuition to purposefully design your simulations with appropriate assumptions at an appropriate stage in the design is critical, as without it – it is just a pile of simulations.
If you would like to know more about how Altair nanoFluidX can be used and how our workflow sets a new standard in the area of drivetrain oiling simulations – please reach out to your nearest Altair representative.