Aircraft Gearbox Development with Simulation-driven Design
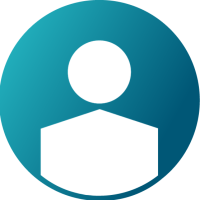
Reducing aircraft development time and cost is critical for all levels of the aerospace industry, from aircraft operators to OEMs to aeroengine manufacturers and aero systems manufacturers.
Developers of aerospace gearboxes in particular are faced with a number of unique challenges. Gearboxes are long lead-time items, so manufacturers are constantly looking for ways to gain engineering insights to help drive performance and noise management improvements, reduce failure risk, reduce component weight, and reduce overall lead time.
The biggest challenges in gearbox design are lubrication and thermal management. Inadequate lubrication or heat management can lead to maintenance issues and failures, while overlubricating can result in unnecessary power loss and higher weight. Decisions driving these complex design strategies, however, are typically arrived at through trial and error and experimentation. With limited time and resources for exploring alternatives, and without the ability to precisely quantify an optimal lubrication solution, gearbox designers typically err towards overlubricating to avoid failures.
Manufacturability is another critical issue gearbox designers face. Casting defects can lead to maintenance and failure issues, but castings are typically designed using experience-based trial and error as well. This iterative process adds significant time and cost to the overall development with each trial and subsequent redesign.
In order to fully understand and optimize the complex systems required for fuel-efficient modern aircraft, aerospace engineers leverage an approach called simulation-driven design (SDD). In this document, we apply an SDD workflow to streamline the development of an aircraft engine gearbox housing, bringing significant cycle time reductions.
The Benefits of Simulation-driven Design
While simulation has long been an important aspect of aerospace design, it is often thought of primarily as a validation tool to verify that a design will meet mission requirements. At the validation stage, designers often have a limited ability to make changes to the initial concept, and the discovery of major performance issues trigger time-consuming redesign iterations. By contrast, a proactive simulation-driven design approach offers engineers simulation insights throughout the development process, giving aero manufacturers greater confidence that their design will meet mission requirements for performance, weight, and manufacturability.
By leveraging an integrated suite of software, gearbox housing designers can use SDD to optimize design performance across multiple disciplines encompassing structures, flexible multi-body dynamics, fluids, thermal management, electromagnetics, system modeling, and embedded systems.
Applying Simulation-driven Design to an Aerospace Gearbox Housing
Gearbox developers are not only challenged to create a robust design; they are also under immense pressure to reduce the design cycle time to drive down cost and time-to-market.
With the goal of efficiently designing a manufacturable gearbox, a simulation-driven design and multiphysics optimization workflow was established to design a lightweight gearbox housing that met performance requirements while minimizing the design time. The major steps of this workflow are as follows.
Gearbox Housing Design Workflow
Gearbox Housing Design Workflow
- Driveline System Design: The first step was to generate a driveline concept to meet the system performance requirements using a Model-based Development approach. A duty cycle was applied to the gearbox housing system model, a simulation that considered its flight modes, mission profile, emergency modes, and airworthiness requirements. Gear and bearing loads were calculated during this step to be used later as an input for computational fluid dynamics (CFD) and structural optimization. System modeling also uncovered the heat generated at the gear mesh; a critical piece of information used to determine the amount of lubricant to inject into the gearbox.
- CAD for CFD Analysis: Based on the driveline concept established in step 1, CAD geometry was generated for the inner mold line of the housing around the gear train. Additionally, CAD geometry was generated for oil inlets and outlets. The target in this step is to create a geometry of the gear housing around the gear assembly designed in the concept development phase. Not meant to be production CAD, this model is developed specifically to be used for CFD and heat transfer simulation, as well for structural analysis and optimization in subsequent steps.
- CFD Simulation: Design studies were conducted with CFD to minimize the amount of oil needed in the gearbox while ensuring adequate lubrication. Design parameters included the number of oil inlets and outlets, location and orientation of oil inlets, inlet velocities, and inner mold line geometry. The target of this step is understanding the oil flow within the gearbox and improve lubrication and heat management with the provided amount of oil. Additional detail on this step can be found in the following section “Spotlight on Oil Flow CFD and Thermal Analysis”.
- Housing Structural Optimization: To ensure a lightweight design that met performance criteria, structural optimization of the aircraft gearbox housing was carried out, starting with the inner mold line geometry. First, topology optimization helped to generate the concept design. The initial topology results were interpretated, then refined by size and shape optimization to design the final housing for structural performance. Thermal loads obtained in previous steps were included in the optimization, including thermal engine loads and thermal loads due to altitude-dependent temperature variation. Design performance targets included, stresses, fatigue life, vibration, and noise.
- Manufacturing Feasibility: Manufacturability assessment was then conducted on the housing. Gearbox housings are typically manufactured using the casting method, so this workflow used casting simulation to visualize potential casting defects such as air entrapment, shrinkage porosity, cold shuts, or mold degradation. Simulation of the manufacturing process allows designers to rectify issues early in the development process, helping to avoid costly downstream corrections.
This workflow focuses on the design of the housing and lubrication for the aircraft gearbox, but it can be further extended to study more simulations such as external CFD, material design study or acoustic analysis. The SDD process used for the gearbox housing can also be applied to concurrent development workflows for the gears and bearings.
Connecting this workflow together is Altair OneTM, a collaborative platform providing web-based access to simulation and data analytics technology plus scalable HPC and cloud resources. Altair One enables sharing of critical design information such as scripts, structural models, and multiphysics analysis results with designers and management throughout the development cycle and across the enterprise. This portal connects engineering data to the broader development process, where users can view and collaborate on work orders, low- and high—order analysis results, trade studies, and more.
Spotlight on Oil Flow CFD and Thermal Analysis
Oil in any transmission mechanism has two primary roles: to provide lubrication and to dissipate heat. Thermal dissipation is especially important in aerospace systems, where great power density poses significant challenges in efficient heat management.
Understanding the flow behavior inside the gearbox is of critical importance for a good design. Previously, the assessment of oil flow behavior and its influence on heat dissipation could only be made through expensive experiments and very limited classic Eulerian CFD methods. No tool, until now, could provide solid or even moderate understanding of a complete oil flow field inside an aerospace gearbox.
In this workflow, Altair CFD tools produced substantial oil flow and thermal results. Here we highlight a few of the key insights from these simulations.
Vortices Inside the Gearbox
Strong vortices can be observed around each of the gears inside the gearbox. This results in very prominent windage effects which could cause non-intuitive oil flow structures.
Time Averaged Oil Distribution
The oil sump is being disturbed in the lower left area of the gearbox. Three inlet jets out of five are also clearly visible in the central and right areas of the gearbox.
Combined Oil-Air Streamlines Coming from The Central Inlet
Streamlines are colored and transparency-weighted for oil volume fraction - more opaque regions reflect more oil. This way, the engineer can see simultaneously where the oil goes and how much of it is there. The red arrows emphasize non-intuitive windage-caused flow behavior. The green arrows indicate rotation direction of each gear. The streamlines can be isolated from each individual inlet or any other specific location.
Steady-State Temperature Distribution on The Gearbox Housing
Two bearings show increased temperatures implying insufficient oil supply at those locations and the need to improve the heat management of the area. Asymmetric temperature distribution can also lead to uneven housing deformation because of thermal stresses, thus introducing potential causes of additional noise and vibration.
Summary of CFD Findings
Insights provided from CFD simulation and thermal analysis enable engineers to improve the heat management of their product by directing the oil to locations where it is needed. For example, modifications of the housing mold, adjust mass flow of the jets, jet positioning, baffle positioning and similar design changes can direct the oil flow to a bearing that appears to be oil-starved and overheating.
The oil flow simulation of the gearbox chosen for this study took 4 days and 9 hours on 8 NVIDIA A100 Tensor Core GPUs for 1.5 seconds of physical time. It took 2 days and 5 hours on 16 NVIDIA A100 GPUs. Altair’s GPU-based smoothed-particle hydrodynamics (SPH) CFD software makes such simulation run times possible. Due to the inherent speed of this approach, designers can iterate on the design variants in order to improve oil supply and heat management, so that by the time a prototype is tested, a solid understanding of its behavior is already established. These simulation times for fully transient, multiphase CFD simulation could not be achieved using classic Eulerian methods in a realistic industrial environment. Further, Altair provides turnkey access to cloud-based high-performance computing resources via Altair One.
Understanding temperature distribution on the housing allows for thermal expansion analysis. Due to usually unequal temperature on the housing, thermal expansion can lead to misalignment of the shafts and the introduction of noise and vibration sources. These CFD insights can be used to achieve more uniform temperature distribution on the housing and mitigate these issues.
Finally, the question of using oil for heat management and lubrication is also related to efficiency, either through a small saving in oil mass needed to perform the tasks of cooling and lubrication or through churning and windage energy losses. CFD simulations can provide valuable information about trends that could impact the design.
Want to learn more? Check out this Webinar
Spotlight on Manufacturability Assessment
It is critical to ensure that a gearbox housing design can actually be manufactured. Late-stage design changes are both costly and disruptive to the manufacturer’s tight development schedule, so insights into manufacturability in the product design stage serve both to drive a more optimal initial design and avoid headaches down the road. In this study, it was assumed that housings were manufactured using casting.
Most casting simulation software requires a highly specialized FEA expert to set up and run. These models take a long time, often several days, to build and set up. Additionally, the analyses typically run for hours to days before delivering results. For ‘what-if’ design studies, this approach would be unfeasible for manufacturers.
This process uses Altair Inspire Cast, which uses advanced approximations to speed up the filling simulation, the most compute intensive step of a casting simulation. This allows one to run the entire casting simulation, including filling and solidification, much faster than in other casting tools. The intuitive Inspire user interface also allows for modeling and problem setup time to be completed in minutes. The process allows designers to perform multiple ‘what-if’ studies to arrive at a manufacturable final design quickly and confidently.
The casting simulation performed consists of two sub-steps:
- Stand-alone solidification analysis
- In-tandem filling and solidification analysis
While in a physical casting, solidification follows filling, but stand-alone solidification analysis was performed first in the simulation workflow to yield faster turn-around time. For a typical casting, filling analysis takes an order of magnitude longer to run than solidification analysis, so engineers can speed up the design process by first running many iterations of stand-alone solidification analysis. The solidification iterations are then followed up by running in-tandem filling and solidification analysis iterations.
Analysis of solidification simulation results help identify and minimize shrinkage defects such as porosity and pipe shrinkage. Solidification modulus, which is the ratio of cooling rate to surface area, is used to identify and resolve shrinkage issues. Higher shrinkage defects are found in regions with high solidification modulus. That issue is resolved by placing risers in the proximity of regions with high solidification modulus. Given the solidification modulus values and location of risers, the software calculates the riser dimensions. This makes the process much easier. Experienced casting designers may analyze time-temperature history to gain further insight into the solidification process to make better design decisions.
Results of solidification analysis
Resolution of some defects may require changes in the casting design. The Inspire user interfaces is designed to make rapid design changes for reanalysis, thus enabling the designer to perform many ‘what-if’ analyses quickly for design exploration.
Modified model for casting-solidification analysis
When the desired solidification performance is achieved, the designer moves to the next step, improving the design by running in-tandem filling and solidification analyses. At this point, the designer may add features such as gates, chillers, sleeves, and coolers as needed. The goal of this step is to identify and minimize defects such as misruns, cold shuts, and last air. These defects are related to the flow of metal and its solid fraction in the casting. The Inspire software provides a time-history of solid fraction within the entire mold. Studying that time-history helps resolve such issues by adding features such as gates, coolers, chillers or by changing part design.
Altair's Work in Aerospace
Altair has been instrumental in pioneering the establishment of optimization centers at major aerospace OEMs. Altair's simulation technologies develop complex, high-fidelity finite-element models for the predictive virtual testing of airframes, engines, and aircraft interiors. We accurately simulate impact damage and correlate against vulnerability events. Now, new methods fuse with old for entirely new processes that take us further than ever into the beyond.
Learn more at https://www.altair.com/aerospace/